Why do we need adaptive optics?
There are two places for optical telescopes: on the surface of the earth and in space. The effort to put a telescope in space and maintain it is much greater than that required for a ground-based telescope, so why would this avenue of observation have ever been pursued? The answer to this question is, simply, the atmosphere. Earth has an atmosphere, which affects light traveling through it.
The variances in the atmosphere consist mainly of differences in temperature from one region to another. The pockets of differing temperatures mean that a path straight from a distant source to a telescope located on the surface of the earth would include regions of higher and lower temperatures? Why does this matter for light?
Photons propagate as waves. The speed of a propagating wave is dependent upon the medium through which it is propagating. For photons from distant stars, the majority of the medium they propagate through is the interstellar medium, which can be approximated as a vacuum (this is what I have been taught, but the fact that Caltech has a whole class on the interstellar medium –Ay 101, which I will take next year – makes me wonder if this is merely a simplistic approximation... anyway, for now I consider space to be roughly a vacuum). Once the photon reaches the atmosphere, however, it is propagating through much denser air. Even this air is not uniformly dense, however: regions of hotter and colder air change the index of refraction for different air pockets, creating what are essentially different mediums.
If you consider the wavefront of light emitted from a distant star, you can see why this would be a problem. When the wavefront reaches the atmosphere, each individual part of the wave front has propagated at the same speed (since the entire wave front has been propagating through the same medium). However, this is no longer true once the photons pass through the atmosphere. Photons that pass through hotter packets of air will propagate at a greater speed than those traveling through cooler air.
You can imagine what happens to the poor wavefront when each photon travels through different temperature air pockets – the poor thing is in disarray by the time it reaches an earth-based telescope! Some areas of the wavefront have travelled faster while other bits have travelled more slowly – what used to be a smooth wavefront is altered by the atmosphere into a much more “squiggly” form.
This is a problem! One solution to this problem is to place your telescope in space, where the wavefronts don’t have to make the perilous journey through the atmosphere. However, in recent years a much more amazing method of avoiding atmospheric distortion has come into being… and this method is adaptive optics!
What does adaptive optics (AO) do?
Adaptive optics corrects for distortions due to the atmosphere in the wavefront. The way that is does is REALLY COOL – I hardly believed it was real the first time I heard about it!
AO uses a deformable mirror. The mirror itself is deformed!!! Now, this mirror is not the mirror that first receives the image. You aren’t deforming the 5 meter mirror at Palomar, for example. Adaptive optics are not a part of the main mirror, but instead an instrument that you can add onto a telescope. The photons are first received by the ‘big mirror,’ as you might expect, but then refracted to the smaller, deformable mirror, which can correct the image.
Basically, AO corrects the image for atmospheric distortion. The goal is that the image received from a ground based telescope using AO would be as good as an image from a space-based telescope.
How do you calibrate AO (find out how air pockets affect the wavefronts)?
The distortions by the atmosphere on the wavefronts are not random. They are very directly caused by the varying temperatures in the atmosphere. So, theoretically, if you could map the air pockets of different temperatures, you could write program to subtract the “noise” caused by said air pockets.
To measure how much correction is needed, AO systems use mainly two methods: a guide star or a laser. Guide stars are those used as references. We already know what we SHOULD get by observing these stars, so if the observed image differs from the expected image, the difference is the interference due to the atmosphere, and can be corrected in subsequent observations.
Lasers are even cooler! The laser used with AO is a sodium laser, which works by emitting a wavelength of light that will excite sodium atoms, which then emit light back to the telescope. This is sort of a fake guide star – it is used in the same way that a real guide star is, but it is much more versatile!
The laser/guide star calibration method is used simultaneously with the observation of whatever source the telescope is studying. That way, corrections are as accurate as possible. As the air pockets change and the wavefront’s “squiggles” change, the corrections to the entire image can be altered (based on whatever the light from the guide star says the change should be) so the image is as accurate as possible.
How does a deformable mirror correct the image?
Firstly – how is a mirror deformed? The method of deformation is pretty cool! I looked at documentation about the PALM-3000, which is an AO system for Palomar, as a case study of how the method works.
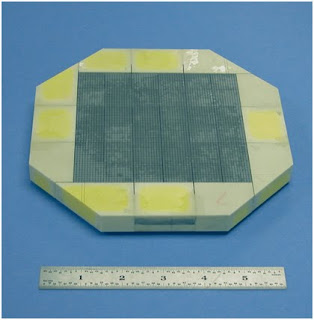
Mirrors are deformed by needle-looking things called actuators. The actuators actually push the surface of the mirror into a pattern that counteracts the effects of the atmosphere. If you don’t think about it too much, the idea that you can just physically counteract the distortions in the wavefront makes sense. Where the wave travelled too slow, push the mirror up so it receives the lagging photons sooner. Where the wave travelled too fast, leave the actuator unpushed so the photons must travel farther and thus all photons arrive at the mirror at the same time. If you start thinking more deeply about this, you will realize how complicated writing the code for this algorithm would be! (Image to the left from 4)
Another remarkable fact is the rate of correction – that is, how often AO reads in data from its guide star and adjusts the actuators to counteract the distortions due to the atmosphere. The whole process sounds computationally arduous – can you believe that AO is corrected 2000 times a second (5)?!? This frequency seems CRAZY to me! Imagine the engineering that went into allowing the fast and precise movements of all those actuators – which, on the PALM 3000, is 4356 actuators (4)!
There are two places for optical telescopes: on the surface of the earth and in space. The effort to put a telescope in space and maintain it is much greater than that required for a ground-based telescope, so why would this avenue of observation have ever been pursued? The answer to this question is, simply, the atmosphere. Earth has an atmosphere, which affects light traveling through it.
The variances in the atmosphere consist mainly of differences in temperature from one region to another. The pockets of differing temperatures mean that a path straight from a distant source to a telescope located on the surface of the earth would include regions of higher and lower temperatures? Why does this matter for light?
Photons propagate as waves. The speed of a propagating wave is dependent upon the medium through which it is propagating. For photons from distant stars, the majority of the medium they propagate through is the interstellar medium, which can be approximated as a vacuum (this is what I have been taught, but the fact that Caltech has a whole class on the interstellar medium –Ay 101, which I will take next year – makes me wonder if this is merely a simplistic approximation... anyway, for now I consider space to be roughly a vacuum). Once the photon reaches the atmosphere, however, it is propagating through much denser air. Even this air is not uniformly dense, however: regions of hotter and colder air change the index of refraction for different air pockets, creating what are essentially different mediums.
If you consider the wavefront of light emitted from a distant star, you can see why this would be a problem. When the wavefront reaches the atmosphere, each individual part of the wave front has propagated at the same speed (since the entire wave front has been propagating through the same medium). However, this is no longer true once the photons pass through the atmosphere. Photons that pass through hotter packets of air will propagate at a greater speed than those traveling through cooler air.
You can imagine what happens to the poor wavefront when each photon travels through different temperature air pockets – the poor thing is in disarray by the time it reaches an earth-based telescope! Some areas of the wavefront have travelled faster while other bits have travelled more slowly – what used to be a smooth wavefront is altered by the atmosphere into a much more “squiggly” form.
This is a problem! One solution to this problem is to place your telescope in space, where the wavefronts don’t have to make the perilous journey through the atmosphere. However, in recent years a much more amazing method of avoiding atmospheric distortion has come into being… and this method is adaptive optics!
What does adaptive optics (AO) do?
Adaptive optics corrects for distortions due to the atmosphere in the wavefront. The way that is does is REALLY COOL – I hardly believed it was real the first time I heard about it!
AO uses a deformable mirror. The mirror itself is deformed!!! Now, this mirror is not the mirror that first receives the image. You aren’t deforming the 5 meter mirror at Palomar, for example. Adaptive optics are not a part of the main mirror, but instead an instrument that you can add onto a telescope. The photons are first received by the ‘big mirror,’ as you might expect, but then refracted to the smaller, deformable mirror, which can correct the image.
Basically, AO corrects the image for atmospheric distortion. The goal is that the image received from a ground based telescope using AO would be as good as an image from a space-based telescope.
How do you calibrate AO (find out how air pockets affect the wavefronts)?
The distortions by the atmosphere on the wavefronts are not random. They are very directly caused by the varying temperatures in the atmosphere. So, theoretically, if you could map the air pockets of different temperatures, you could write program to subtract the “noise” caused by said air pockets.
To measure how much correction is needed, AO systems use mainly two methods: a guide star or a laser. Guide stars are those used as references. We already know what we SHOULD get by observing these stars, so if the observed image differs from the expected image, the difference is the interference due to the atmosphere, and can be corrected in subsequent observations.
Lasers are even cooler! The laser used with AO is a sodium laser, which works by emitting a wavelength of light that will excite sodium atoms, which then emit light back to the telescope. This is sort of a fake guide star – it is used in the same way that a real guide star is, but it is much more versatile!
The laser/guide star calibration method is used simultaneously with the observation of whatever source the telescope is studying. That way, corrections are as accurate as possible. As the air pockets change and the wavefront’s “squiggles” change, the corrections to the entire image can be altered (based on whatever the light from the guide star says the change should be) so the image is as accurate as possible.
How does a deformable mirror correct the image?
Firstly – how is a mirror deformed? The method of deformation is pretty cool! I looked at documentation about the PALM-3000, which is an AO system for Palomar, as a case study of how the method works.
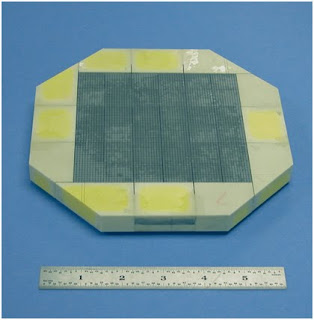
Mirrors are deformed by needle-looking things called actuators. The actuators actually push the surface of the mirror into a pattern that counteracts the effects of the atmosphere. If you don’t think about it too much, the idea that you can just physically counteract the distortions in the wavefront makes sense. Where the wave travelled too slow, push the mirror up so it receives the lagging photons sooner. Where the wave travelled too fast, leave the actuator unpushed so the photons must travel farther and thus all photons arrive at the mirror at the same time. If you start thinking more deeply about this, you will realize how complicated writing the code for this algorithm would be! (Image to the left from 4)
Another remarkable fact is the rate of correction – that is, how often AO reads in data from its guide star and adjusts the actuators to counteract the distortions due to the atmosphere. The whole process sounds computationally arduous – can you believe that AO is corrected 2000 times a second (5)?!? This frequency seems CRAZY to me! Imagine the engineering that went into allowing the fast and precise movements of all those actuators – which, on the PALM 3000, is 4356 actuators (4)!
Why is AO useful?
As they say, a picture is worth one thousand words. This Palomar image shows the difference between an observation with adaptive optics (right) and an observation without (left) (image from 2).
Useful links:
1. http://www.mtwilson.edu/ao/ - tells about the AO system used at Mt. Wilson; good explanation about AO more generally as well
2. http://www.astro.caltech.edu/palomar/AO/ - cool images – AO is important at Palomar!
3. http://amazing-space.stsci.edu/resources/explorations/groundup/lesson/basics/g18a/ - good image on what the air pockets do
4. http://spie.org/x39226.xml?ArticleID=x39226 – specifically about the PALM-3000, good explanation on how the actuators work and some cool images!
5. http://www.oir.caltech.edu/twiki_oir/bin/view/Palomar/Palm3000/WebHome - more on PALM-3000
This is an absolutely outstanding writeup of the importance of AO in astronomy. Your description of the wave fronts' perilous journey through the Earth's atmosphere is spot on and very easy to visualize and understand.
ReplyDeleteThanks for going above and beyond with your post-Ay20 blogging. Excellent job!
I was able to follow this and found it very understandable and interesting even though I knew nothing about the topic before. Thank you for writing this!
ReplyDelete